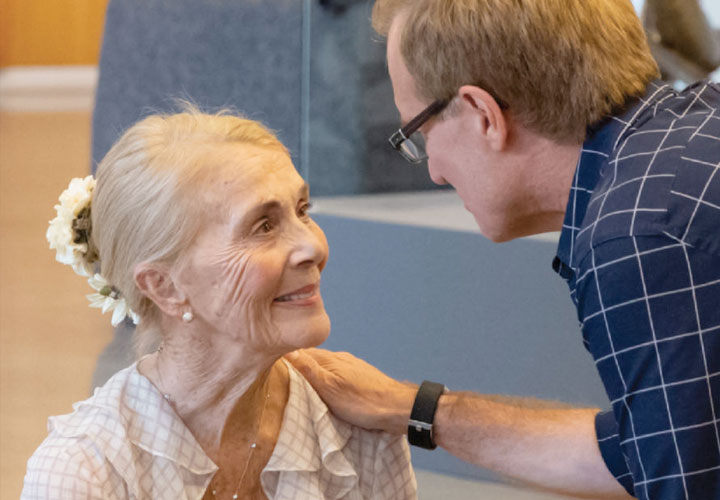
2018 was a year of exciting change at ISB.
Following our affiliation with Providence St. Joseph Health in 2017, Dr. Lee Hood stepped down as ISB president to focus on his role of chief science officer and senior vice president at PSJH. Dr. Jim Heath, a renowned scientist who has a long history with ISB, was named our new president, and immediately took on the role with an eye on the future.
Our 2018 Annual Report features a president’s letter from Dr. Heath that addresses some of the highlights of 2018 and offers a glimpse at what is coming in the very near future; an acknowledgement of the generous contributors who help fund the groundbreaking scientific research at ISB; a collection of our top news stories of the year; and a snapshot of ISB’s finances.
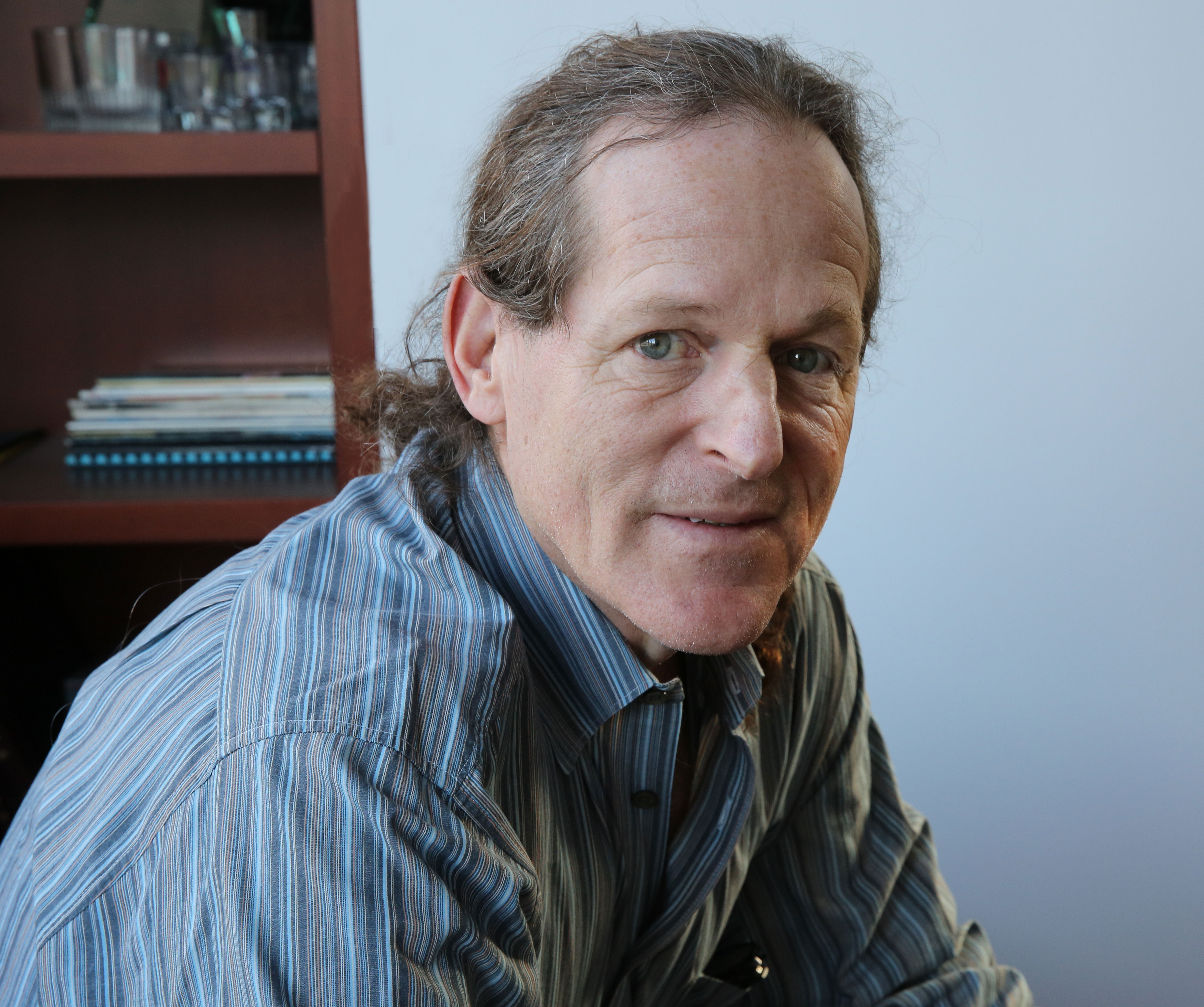
From the desk of Dr. Jim Heath
Letter from the President
I proudly took over the role of ISB president on January 1, 2018. The mantle was passed to me by Dr. Lee Hood, a legend in the field of genomics and biology and ISB’s co-founder.
Since our inception in 2000 as the world’s first systems biology-focused institute, we have championed collaborative and cross-disciplinary science. These values are as important today as they were 19 years ago.
Furthermore, translational science -- quickly moving scientific breakthroughs from concept to clinic -- is a critical part of ISB’s mission, and one we are attaining thanks to our affiliation with Providence St. Joseph Health (PSJH), one of the largest not-for-profit health care providers in the U.S.
In 2018, we expanded our faculty with the addition of myself and Drs. Sean Gibbons, Jenn Hadlock, and Wei Wei. We have continued to play an important role with STEM education by working to ensure all students have access to quality education and by developing next-generation STEM curricula on behalf of teachers. We created the ISB Foundation Board to help support ISB’s research and education programs through philanthropy, and we wished a very happy 80th birthday to Lee Hood.
It was an exciting year to be sure, and 2019 continues that trend. We are fearlessly pursuing research areas where our scientists are uniquely positioned to make a difference, including cancer immunotherapy, scientific wellness, brain health, Lyme disease, and many more. We have also begun working with the PSJH system to incorporate some of the concepts of systems biomedicine to help evolve PSJH into a state-of-the-art learning health care system designed to improve health outcomes for all of its patients.
As we push forward in this new chapter in the life of ISB, we are excited to unveil a new brand and tagline. (You can see our new logo and branding elements on this Annual Report!) Ahead of our 20th anniversary in 2020, we felt it was time to re-introduce ISB to our community and the world. “Science Transforming Health:” These three words perfectly sum up our mission to change lives with our important work.
You will be seeing a lot more of ISB going forward. As part of our year-long anniversary celebration in 2020, we are partnering with Town Hall Seattle to put on a speaker series focusing on some of the most exciting topics in science. Additionally, we will have several ISB-hosted events where the public can come meet our scientists, learn about ISB, and help us change the world.
ISB has always been a problem-solving institute. We are proud of our storied past, and we look forward to a limitless future.
Sincerely,
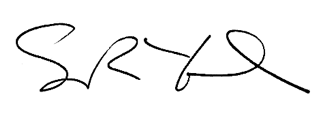
James R. Heath, PhD
President, ISB
Financials
For the Year Ending December 31, 2018
Revenues
Statement of Activities
Dollars in Thousands
Revenues | $ |
% |
---|---|---|
Grants & Contract Revenue | 21,888 |
80.1 |
Contributions | 3,391 |
12.4 |
Investment & Other Income | 2,039 |
7.5 |
Total Revenues | 27,318 |
100.0 |
Expenditures | $ |
|
Research & Other Direct Costs | 24,036 |
|
Management & General | 12,269 |
|
Fundraising & Other | 319 |
|
Total Expenditures | 36,624 |
|
Decrease in Net Assets | (9,306) |
Balance Sheet
Dollars in Thousands
Assets | $ |
---|---|
Cash & Investments | 56,673 |
Other Assets | 7,305 |
Property & Equipment (Net) | 10,200 |
Total Assets | 74,178 |
Liabilities | $ |
Accounts Payable & Accrued Expenses | 8,073 |
Deferred Revenues | 3,038 |
Total Liabilities | 11,111 |
Net Assets | $ |
Net Assets without Donor Restrictions | 47,889 |
Net Assets with Donor Restrictions | 15,178 |
Total Net Assets | 63,067 |